Bacterial community dynamics, co-occurrence relationship and assembly processes associated with two Acropora corals in nursery transplantation
-
Abstract: Coral gardening has become a promising technique for restoring reefs worldwide in the Anthropocene era. The microbiome plays an important role in enhancing adaptive resilience in situ nursery propagation of corals. However, little is known about the response patterns of bacterial community dynamics, co-occurrence networks and assembly processes of different species in coral restoration nurseries over time. Here, we collected two Acropora coral samples from transplanted fragments and source colonies at 1- and 3-month post-transplantation (May and July 2022) in an upwelling-affected fragmented reef. Full-length 16S rRNA gene sequencing revealed that bacterial communities of coral fragments in nurseries exhibited consistent temporal shifts compared to those of the source colonies. High host specificity was observed in the bacterial community and network structure associated with source colonies. In contrast, for the two coral species within nurseries, there were no differences in bacterial diversity, composition and core microbiome. Stochastic assembly processes were identified as the primary drivers of bacterial communities in all May samples, whereas deterministic processes played a more prominent role in July. Seawater properties (e.g., temperature and ammonium concentration) partially explained the compositional changes in the bacterial communities of these coral samples. Our findings suggested that coral nurseries contributed to the homogenization of bacterial communities in different Acropora corals, despite the apparent temporal dynamics of bacteria. These results enhance our understanding of the coral microbiome, ecological interactions and assembly principles in different host within in situ nurseries.
-
Key words:
- In situ nursery /
- Coral transplantation /
- Bacterial community dynamics /
- Host specificity /
- Similar assemblages /
- Environmental adaptation
-
1. Introduction
Coral reefs are one of Earth's most biodiverse and productive ecosystems, providing a wide range of goods and services (Moberg and Folke, 1999; Ramesh et al., 2020). However, coral reefs coverage worldwide is declining precipitously due to both global and local stressors (Hughes et al., 2017). Given the ongoing impacts of climate change and human activities, conventional management is proving to have limited effectiveness for reef recovery (Rinkevich, 2021; Williams et al., 2019).Thus, there is a growing recognition that local active management approaches are needed to assist reef recovery (Rinkevich, 2021). In particular, coral gardening has emerged as an active and innovative management approach that involves transplanting live coral fragments onto suitable underwater nurseries (Abrina and Bennett, 2021). Asexual reproduction through in situ coral nurseries has been widely employed as a quick cloning strategy for coral restoration (Ramesh et al., 2020). Overall, coral gardening is a successful, low-technology, and low-cost restoration technique for increasing coral cover and restoring degraded reefs (Strudwick et al., 2022). However, the success of coral fragments in nursery is subject to considerable variability and influenced by multiple factors, including attachment substrate, water quality, coral characteristics, and transplant methods (Smith et al., 2019).
The holobiont is the complex assemblage constituted by the coral host and the associated microorganisms (Deignan and McDougald, 2022; Kanisan et al., 2023). Coral-associated microbial communities play crucial roles in promoting the health and resilience of corals (Bourne et al., 2016; Peixoto et al., 2017), encompassing critical functions such as energy provision, nitrogen fixation, and protection against pathogen (Beatty et al., 2022; Miller et al., 2020). Numerous studies have reported that the composition of coral-associated bacterial community is strongly influenced by both environmental factors and host identity (Damjanovic et al., 2020; Ziegler et al., 2019). For example, bacterial community associated with Acropora hemprichii is highly flexible in response to environmental disturbances, whereas Pocillopora verrucosa exhibits more stable microbiomes even under changing environmental conditions (Ziegler et al., 2019). Recently, increased research effort has been devoted to comprehensively characterize coral microbial communities in response to climate change and understand their potential to withstand environmental perturbations (Deignan and McDougald, 2022; Webster and Reusch, 2017). Given the critical role of microbial communities in the fitness of their hosts, manipulation of coral probiotic strains has recently been proposed to improve restoration efforts and mitigate the loss of coral reefs (Miller et al., 2020). Consequently, it is imperative to thoroughly investigate the environmental drivers and limitations of microbial flexibility in coral fragments within in situ nurseries to ensure the effectiveness and success of coral propagation procedures (Strudwick et al., 2022).
Gaining a comprehensive understanding of the dynamics in coral microbiome across coral species can greatly aid restoration efforts, as they play pivotal roles in coral plasticity and holobiont adaptation (Aguirre et al., 2022). For instance, recent studies have found potential differences in the flexibility of bacterial associations across environmental gradients, as demonstrated by reciprocal transplantation designs (Strudwick et al., 2022; Ziegler et al., 2019), where coral fragments from pristine sites are transplanted into novel reef environments (Deignan and McDougald, 2022; Ziegler et al., 2019). However, the response of the microbial community associated with transplanted corals to nurseries has often been overlooked, severely limiting the optimization of coral transplantation as a reef restoration effort (Strudwick et al., 2022). Additionally, information on the species coexistence and assembly mechanism of bacterial community within transplanted colonies is limited. Hence, we aimed to address this knowledge gap by studying coral fragments in nurseries over small geographic scales and determining the extent of host type and propagation process in maintaining bacterial assemblages over time. More specifically, our objectives were to address the following questions: (1) Whether bacterial communities associated with Acropora corals exhibit high host specificity and temporal dynamics in nursery fragments and source colonies? (2) What are the patterns of interaction network and community assembly in these different bacterial communities? Our findings can serve as a fundamental basis for elucidating the relationships between coral microbiome flexibility and environmental adaptation potential within propagation nurseries.
2. Materials and methods
2.1 Transplantation experiment and samples collection
The study site, Fenjiezhou (FJZ) Island, is located in the South China Sea off the east coast of Hainan Island, known for its popularity among recreational divers and tourism development (Zhu et al., 2023a). The northwest area of FJZ Island, specifically at coordinates N18°34′ and E110°11′, has been significantly impacted by increasing human disturbance, resulting in noticeable degradation of the coral reefs, thus necessitating urgent artificial restoration efforts (Fig. S1). The nursery (patent publish number: ZL202210374323.1) used in this project is a new and cost-effective tool for coral restoration (Liu et al., 2024). Branching corals in the genus Acropora have been heavily favored in transplantation experiments due to their high potential of asexual reproduction and reattachment capacity. The project work commenced on April 19, 2022, and was completed on April 24. A total of 52 nurseries were constructed in the degraded area (on the sand and rocky base) immediately adjacent to the reef, and 628 Acropora hyacinthus and Acropora microphthalma fragments were transplanted (Fig. 1). All Acropora fragments in coral nurseries were randomly selected and originally collected from source colonies on the reef. Coral branches were fragmented from a maximum of one quarter of each colony, and cable ties were employed to secure the fragment to the nursery frame (Fig. S2).
Figure 1. Experimental design and sampling timeline during the nursery phase. In-situ coral nursery frame that transplanted coral fragments of Acropora hyacinthus and Acropora microphthalma were built in the degraded area adjacent to the reef in Fenjiezhou Island. Acropora fragments were originally collected from source colonies on the reef, and then each fragment was based to the nursery frame using a cable tie. For a sampling event (in May and July) during of the experiment, six small partial fragments of each species were obtained from the coral nursery and the six corresponding source colonies of each species were sampled.Vertical projection photos of each transplanted coral fragment in the nursery were taken using an underwater camera (Olympus-TG4) and a ruler during the surveys, conducted after 1 month (May) and 2 months (June) of transplantation. The survival, mortality and growth rate of transplanted corals were calculated based on these photos (Liu et al., 2024). Specifically, orthographic projection area of the living part of each coral fragment was determined in these photos with a ruler inside using ImageJ software. The growth rate of each fragment was calculated as difference between the final area and the initial area divided by the transplantation months. Meanwhile, we used the photos to determine the survival based on the absence of living tissue or loss of coral. In addition, in May and July 2022 (1- and 3-month post-transplantation), six replicate of visually healthy fragments (~5 cm2) of the two Acropora species were randomly collected from transplants in coral nurseries and source colonies on the reef. Finally, 48 visually healthy coral samples (2 species
$\subseteq $ 2 types$\subseteq $ 2 months$\subseteq $ 6 replicates) were selected using self-contained underwater breathing apparatus diving (SCUBA) with diagonal pliers, and they were then stored at −20℃ in sterile centrifuge tubes.In April 2022, a HOBO water temperature logger (UA-002-08) was deployed on a coral nursery in the experimental area. General environmental parameters and dissolved nutrients concentrations within 1 m of the transplant frames and reefs were determined on each sampling day according to methods described in our previous study (Zhu et al., 2023b). Specifically, we used a water quality multiprobe (Eureka Water Probes, USA) to record the seawater temperature, salinity, and dissolved oxygen (DO), then measured turbidity and depth using an AQU Alogger 210 (Aquatec, United Kingdom). Water Samples (100 mL) were filtered (Whatman GF/F, 0.45 μm, United Kingdom) and the inorganic nutrients was analyzed by an automatic nutrient analyzer (Seal AA3, Germany).
2.2 DNA extraction and full-length 16S rRNA gene sequencing
For all coral samples, the entire sampled fragment was crushed in liquid nitrogen. DNA was extracted using the DNA extraction kit following the manufacturer’s instructions. DNA concentration was measured using a nanodrop 2000 spectrophotometer (bio rad Laboratories Inc., USA.). The 16S rRNA full-length gene amplification amplicons were amplified using the primers 27F (5′-AGGRGTTYGATYMTGGCTCAG-3′) and 1492R (5′-RGYTACCTTGTTACGACTT-3′) for bacterial communities. PCR amplifications were performed in triplicate for each sample using the following conditions: an initial denaturation at 95℃ for 30s, followed by 35 cycles each with 30 s at 95℃, 30 s at 60℃, and 45 s at 72℃, and a final extension of 10 min at 72℃. According to the standard protocol of Majorbio biopharm Technology Co., Ltd. (Shanghai, China), purified amplicons were used for sequencing on the Pacbio Sequel II System (Pacifc Biosciences, USA). The PacBio raw reads were processed and sequences after quality filtering sharing 97% similarity were clustered into Operational Taxonomic Units (OTUs) as previously described (Zhu et al., 2023). The taxonomy of OTUs was assigned against the Silva database (v138) by the RDP classifier.
2.3 Statistical analysis
The differences of environmental parameters between different locations from the same season or different seasons from the same location were analyzed by Student’s t-test. The independent sample t-test was performed on the growth rate of the same season in different species or the same coral in different seasons. Results were expressed as mean ± standard error at a significance level of p < 0.05.
In the downstream analysis, OTU normalization was performed by rarefying the sequencing depth of the sample with the fewest sequences. Shannon index was calculated using the “vegan” package in R v4.02 to assess the diversity of bacterial communities. To examine differences in the Shannon index among different groups, analysis of variance (ANOVA) was used after verifying homogeneity of variance and normality assumptions. In addition, canonical analysis of principal coordinates (CPCoA) plots and permutational multivariate analyses of variance (PERMANOVA) utilizing Bray-Curtis dissimilarity matrices were employed to examine differences in coral-associated bacterial community structure across coral species and sampling types over time. In the study, p-values < 0.05 were considered statistically significant. In addition, members of the coral core microbiomes, present in at least 95% of all coral samples regardless of their relative abundance, were identified (Osman et al., 2020).
Co-occurrence networks were inferred based on the Spearman correlation matrix constructed with correlation coefficients |r| ≥ 0.6 and p-values < 0.01, and OTUs with detectable or low average relative abundance (less than 0.1% in data sets) were removed in the co-occurrence network. The topological characteristics were calculated using the “igraph” package, and the networks were visualized using Gephi software. A neutral community model (NCM) was used to estimate the effects of stochastic processes on bacterial community assembly by fitting the relationship between the frequency of OTUs occurrence and their relative abundance (Sloan et al., 2006). In this model, R2 indicates the goodness of fit to the NCM. To quantify the relative importance of deterministic and stochastic processes in community assembly, we further calculated the modified stochasticity ratio (MST), with 50% as the threshold between more deterministic (MST < 50%) and more stochastic (MST > 50%) assembly (Ning et al., 2019).
3. Results
3.1 Water quality, coral samples and sequencing overview
The results from the HOBO temperature record revealed that the seawater temperature at FJZ Island decreased from June to July 2022, which was attributed to the influence of Qiongdong upwelling (QDU) (Fig. S3). Detailed information about in-situ water quality variables of the study can be found in Fig. S4. Moreover, the concentrations of ammonium and dissolved oxygen were significantly higher in May compared to July (p < 0.05), with values changing from 8.573 ± 0.264 to 4.569 ± 0.213 μmol/L and 7.685 ± 0.013 to 6.78 ± 0.074 mg/L, respectively. Seawater nitrate, phosphate, silicate concentrations, and turbidity showed no significant changes between the two months. Most environmental conditions in the same month had no difference between nursery structure and reef site. Overall, the water quality parameters were more influenced by seasonal dynamics rather than regional properties, as most parameters in the source and transplant areas exhibited similarities.
Transplanted coral survival and growth rate were monitored and analyzed (Fig. S5). In May, 1 month after transplantation, A. hyacinthus (AH) fragments exhibited a high survival rate of 99.79% (with only 1 out of 483 fragments showing mortality), while the survival rate of A. microphthalma (AM) was slightly lower at 97.9% (with 3 out of 143 fragments showing mortality). However, in June, 2 months after transplantation, the survival rate of AH and AM decreased to 99.38% (with 3 out of 482 fragments showing mortality) and 95% (with 7 out of 140 fragments showing mortality), respectively. After transplantation, both AH and AM showed higher growth rates in June (2.49 ± 0.26 and 1.04 ± 0.02 cm2·month−1) compared to May (3.70 ± 0.24 and 1.18 ± 0.02 cm2·month−1), but the growth rate of AH was significantly higher than that of AM in both months (p < 0.05).
Coral sampling was conducted in May and July for bacterial sequencing analysis, including two coral species from nursery fragments and source colonies. Full-length 16S rRNA genes were amplified from coral-associated bacterial communities of 48samples. A total of 2,331,052 clean reads were obtained for all samples, with an average length of 1,488 bp and ranging from 22,493 to 73,352 reads per sample. After rarefying to 22,493 sequences per sample for downstream analysis, rarefaction curves were approaching saturation (Fig. S6), indicating that most bacterial communities were recovered in the sequencing analysis.
3.2 Bacterial diversity
The Shannon diversity of AH from source colonies and transplanted AM samples showed significant variation among collection times (p < 0.05), with lower values occurring in July for both groups (Fig. 2). Differences in bacterial diversity between AH and AM were more significant in the source colonies, with Shannon diversity of AH being much higher than that of AM in May, while Shannon diversity was significantly lower in AH compared to AM in July (p < 0.05). However, there were no significant changes in Shannon diversity for transplants within each coral species during the experiment (p > 0.05), indicating that the bacterial diversity of transplanted AH and AM fragments showed similar patterns in response to the transplantation effect.
3.3 Coral-associated bacterial community structure and composition
CPCoA revealed that the whole bacterial communities tended to cluster together based on different months (p = 0.001) rather than coral species or sampling types (Fig. 3a). Furthermore, the sampling types were found to significantly influence the formation of bacterial communities in May between the two coral species, accounting for up to 19.3% of the overall variance in the data (Fig. 3b). There were significant differences in the community composition between the transplants and source colonies of both A. hyacinthus and A. microphthalma in May (p < 0.05). Notably, the species specificity of the bacterial community structure in transplanted coral fragments in May (AH vs AM: p > 0.05) was considerably lower compared to the natural condition (AH vs AM: R2 = 0.187, p < 0.05). There were no significant changes in the community composition of the transplants compared to the source colonies in both A. hyacinthus and A. microphthalma in July (Fig. 3c), suggesting that the bacterial communities of transplanted fragments stabilized and resembled those of the source colonies after 3 -month transplantation.
Figure 3. CPCoA (Bray–Curtis) plot of bacterial associations within coral host habitats of two Acropora corals (a). The profiles of bacterial community associated with two Acropora corals in May (b) and July (c) between nursery fragments and source colonies. Bacterial community composition (relative abundances) at the family level of nursery fragments and source colonies in May and July (d).The relative abundance of dominant bacterial families including Moraxellaceae, Nocardiaceae, Propionibacteriaceae, and Enterobacteriaceae significantly decreased, while the families Comamonadaceae, Burkholderiaceae, and Xanthobacteraceae exhibited a significant increase from May to July (Fig. 3d). After 1 month of transplantation experiment in May, the abundance of the dominant families in transplanted coral fragments of AH showed no significant changes compared to AM. In July, there was no difference in the abundance of dominant families between transplanted corals and source colonies in both AH and AM. Our results indicated that transplantation promoted compositional homogeneity of the bacterial communities associated with the two coral species, as evidenced by consistent patterns observed in different monthly monitoring periods.
3.4 Coral core microbiome
To investigate the potential existence of a core microbiome, we considered all bacterial OTUs present in at least 95% of all samples. We identified 13 consistently present bacterial OTUs as members of the core microbiomes. Interestingly, significant temporal changes in the assemblage structure of the core microbiome were observed, but no differences were observed in terms of species or source (Fig. 4a). Specifically, the relative abundance of Acinetobacter sp. (OTU3324 and OTU3420), Raoultella sp. (OTU2777, OTU3438, and OTU2780), and Pseudomonas (OTU2770) significantly decreased, while the relative abundance of Ralstonia (OTU3390 and OTU3438), Brevundimonas sp. (OTU3329 and OTU3358), Stenotrophomonas (OTU3309, OTU3248), and an OTU belonging to Bradyrhizobium sp. (OTU3358) was significantly increased from May to July (Fig. 4b).
3.5 Bacterial interaction network
To evaluate the interaction patterns of bacteria associated with corals, we constructed co-occurrence networks by analyzing correlation relationships among different groups (Fig. 5a). The topological properties of these networks were summarized in Fig. 5b to provide a comprehensive description of the intricate interrelationships. Overall, the complexity of all networks, except for AM-S, decreased from May to July, as evidenced by the reduction in the number of edges, nodes, and average degree. Notably, the network structure in AM natural populations displayed a higher connectivity level than AH throughout the experiment. Consistent with the changes in network size (i.e., number of edges and average degree), the robustness of AH-T (0.34) and AM-T (0.33) was comparable in July. However, the robustness of all networks, except for AH-S, decreased from May to July.
Figure 5. Co-occurrence networks (a) and topological properties (b) of coral microbiomes for two Acropora corals collected from nursery fragments and source colonies in May and July. Node color represents different abundant bacterial OTUs within each network. The size of the nodes indicates the number of connections (degree) for each node. Connection between two nodes represents correlations, positive and negative correlations are shown in blue or red lines, respectively.3.6 Bacterial community assembly processes and environmental drivers
To investigate the contribution of stochastic processes to community assembly, we fitted the bacterial communities to Sloan's neutral model (Fig. 6a). The neutral model best described the composition of samples in May, with R2 values ranging from 0.135 to 0.535. However, the fit of the bacterial communities from July to the neutral model was poor, with R2 values less than 0 in all four groups. To further quantify the relative contributions of deterministic and stochastic processes in bacterial community assembly, we employed the MST (Fig. 6b). The results revealed that except for AM-T (with a mean MST of 41.44%) in May, bacterial communities were primarily governed by stochastic processes (with mean MSTs ranging from 55.42% to 70.75%). In contrast, the bacterial community in July exhibited a higher contribution of deterministic processes (with mean MSTs ranging from 11.48% to 36.67%). These findings indicated that the significant assembly processes of bacterial communities differed significantly between May and July.
Figure 6. Fit of the neutral community model (a) and comparison of modified stochasticity ratio (b) showing ecological processes that shape the bacterial community assembly for two Acropora corals collected from nursery fragments and source colonies in May and July. The solid orange line is the best fit to the neutral community model, and the dashed orange line indicates 95% confidence intervals around the model prediction. OTUs that occur more or less frequently than predicted by the model are shown in grey and brown, respectively. R2 represents the goodness of fit to the model. Redundancy analysis (RDA) of coral-associated bacterial community dynamics with environmental variables (c).Further, the RDA analysis (Fig. 6c) was used to explore the main environmental drivers for bacterial composition associated with A. hyacinthus (AH) and A. microphthalma (AM). The overall results of RDA were significant for both bacterial communities of AH (F = 2.299, p = 0.001) and AM (F = 1.985, p = 0.001). The selected environmental variables could explain 44.80% and 39.56% of the overall bacterial composition variations, respectively. Temperature and ammonium had significant effects based on the 999 permutations of the Monte Carlo test (p < 0.01). The Mantel test (Fig. S7) further confirmed that the temperature and ammonium exhibited the strongest relationships with the bacterial communities of two Acropora corals (r > 0.4, p < 0.001).
4. Discussion
Understanding the response mechanisms of coral environmental flexibility with a microbial perspective is critical to reveal the adaptive potential to in situ transplantation. The response of coral-associated bacterial communities to restoration nurseries and the underlying factors driving these changes, especially for interactional networks and community assembly mechanisms, are poorly understood. Through a series of analyses, we have provided a detailed characterization to gain insight into how the coral bacterial communities of different species respond to nursery environments over time. To the best of our knowledge, this study was the first to explore the dynamic changes of the coral microbiome between nursery transplants and source colonies, especially the ecological drivers of ecological networks and assembly processes. The results of this study significantly enhance our understanding of coral microbiome flexibility of nursery-planting fragments.
4.1 Short-term assessment of in situ coral transplantation
Globally, active restoration techniques involving the transplantation of live coral fragments have been developed to facilitate the recovery of damaged reef habitats (Rinkevich, 2005, 2021). In this study, coral fragments from two coral species (A. hyacinthus and A. microphthalma) were transplanted to the nursery in FJZ, an area influenced by Qiongdong upwelling (QDU) and anthropogenic disturbance (Zhu et al., 2022a). Ammonium concentrations were significantly higher than nutrient thresholds reported for healthy coral reefs (Houk et al., 2022). However, a remarkable survival rate (≥ 95%) of the transplanted corals was observed in two underwater surveys. The average survivorship was consistent with the previous study in Wuzhizhou island of Qiongdong upwelling zone (Xia et al., 2022). One possible explanation for this result was that the open structures utilized in the experiment might have provided crucial support for the transplanted coral fragments, allowing relatively unimpeded water flow (Williams et al., 2019). This could have served as a vital nursery tool for cultivating specific coral species with stress resistance through phenotypic plasticity (Rinkevich, 2021). The results revealed that the growth rate of A. hyacinthus was significantly higher than A. microphthalma, consistent with previously observed transplantation effects (Ramesh et al., 2020; Xia et al., 2022). Furthermore, both A. hyacinthus and A. microphthalma exhibited higher growth rates in June than May, suggesting that the upwelling environment might be a potential driver of temporal-specific growth rates. This inference was supported by previous studies demonstrating the positive influence of increased nutrient and organic matter availability resulting from terrestrial input and upwelling on coral growth (Randall et al., 2020; Zhu et al., 2023b).
4.2 Transplanted fragments from nursery have similar bacterial diversity between coral species
Previous studies have demonstrated that coral morphology and host trait significantly determine the high species-specific diversity of coral-associated bacteria (Morrow et al., 2022; Ricci et al., 2022). Bacterial diversity of A. hyacinthus from source colonies and transplanted A. microphthalma were lower in July compared to May in this study, which was consistent with the finding that high water temperature during the summer months leads to lower alpha diversity of coral-associated bacteria (Zhu et al., 2023b). The alpha diversity showed no difference in the transplanted fragments between A. hyacinthus and A. microphthalma over time, while Shannon index of source colonies showed the opposite trend. Despite the species-specificity of the coral microbiome, specific biotic and abiotic factors that vary from nursery frames to the source reef are likely drivers of differences in coral bacterial communities (Strudwick et al., 2022). In particular, the transplanted fragments were affixed to artificial reefs constructed using similar materials and architectural complexity. On the contrary,, the source colonies grew on natural coral reefs with distinct benthic assemblages and structural complexities. The geographic location and water quality parameters were similar, but it was necessary to note that the nursery might not have provided identical attachment conditions for corals compared to the surrounding reef environment. These findings supported the concept of local acclimatization through coral gardening over small geographic scales.
4.3 Temporal dynamics and host specificity of bacterial community structure and core microbiome
The distinct temporal dynamics of bacterial community structure observed in this study suggested that the coral microbiome was flexible and capable of rapid adaptation to changes, which might facilitate adaptation of the source colonies or transplanted corals to stressful environments (Reshef et al., 2006; Ziegler et al., 2019). Furthermore, bacterial communities of transplanted corals still exhibited significant differences from donor colonies after 1 month of nursery planting in May; whereas by July, they had developed similar bacterial assemblages. Other factors such as benthic assemblage, fragmentation of the natural reef, and nursery frames, might drive bacterial differences (Strudwick et al., 2022). Consistent with our results, microbial communities of Pocillopora acuta became similar to those of the local corals at the destination reef within one day and two days post-transplantation (Deignan and McDougald, 2022), while bacterial communities of A. millepora fragments changed to more closely resemble source colonies within 30 days of nursery-planting (Strudwick et al., 2022). In comparison with previous studies that revealed a high degree of host specificity from the transplanted fragments (Strudwick et al., 2022; Ziegler et al., 2019), we found similar bacterial community between transplanted A. hyacinthus and A. microphthalma. These thus highlight that nurseries can contribute to the establishment of similar bacterial communities over time and reduce species-specific differences in bacterial community dynamics compared to the natural reef environment. Moreover, coral can undergo shifts in microbial composition without succumbing to dysbiosis (Deignan and McDougald, 2022). For example, although bacterial families (Moraxellaceae, Comamonadaceae) were more abundant in the affected corals, they can also be isolated from healthy corals (Ziegler et al., 2019). Accordingly, shift in the symbiotic bacterial composition does not exclusively reflect a pathobiome and may contribute to coral holobiont plasticity for withstanding environmental fluctuations (Ziegler et al., 2017, 2019).
The presence of core microbiomes indicates the crucial functional role they play for the host (Zanotti et al., 2020). Significant temporal changes were observed in the core microbiome, but no differences were found based on coral species or transplant treatment. Bacterial groups such as Acinetobacter, Pseudomonas, Bradyrhizobiaceae, Raoultella (Enterobacteriaceae) have commonly been found in coral core microbiomes (Kellogg, 2019). Acinetobacter, a gamma-proteobacteria, has been reported in both healthy and diseased corals, with members of this genus potentially contributing to pathogen defense (Brener-Raffalli et al., 2018; van Bleijswijk et al., 2015). The genus Pseudomonas, known to be part of the coral-associated beneficial core microbiome in the QDU zone, plays an important role in nutrient cycle metabolism and production of anti-microbial compounds (Hernández-Zulueta et al., 2022; Zhu et al., 2023b). Ralstonia has been reported as a core microbiome member in both zooxanthellate and azooxanthellate corals, associated with functions like nitrogen fixation, transport, and amino acid metabolism (Ainsworth et al., 2015; Engelen et al., 2018). Despite the genus Stenotrophomonas has a high abundance in the diseased corals from eutrophic waters (Cárdenas et al., 2012; Lee et al., 2012), it also is the dominant genus in the apparently healthy stony and soft corals (Hernández-Zulueta et al., 2022). Bradyrhizobium is widely reported as nitrogen-fixing bacteria in coral holobiont and may assist its hosts by fixing nitrogen (Ceh et al., 2013; Lema et al., 2014). Therefore, the observed changes in the core microbiomes between May and July in our study further suggested the reasonable flexibility of the coral microbiome in response to environmental changes (Aguirre et al., 2022). Taken together, the coral fragments planted in the nursery did not exhibit a distinct reduction in putatively beneficial core microbiomes compared to the source colonies on the reef (Strudwick et al., 2022), which have been suggested to provide essential functions that allow coral to thrive.
4.4 Co-occurrence patterns and assembly mechanisms of bacterial communities
Network analyses can provide a comprehensive understanding of the ecological interactions among microbiome members within the coral holobiont (Leite et al., 2018a; Lima et al., 2020). Our results indicated that the network complexity and robustness decreased from May to July, because sensitive network structures in the QDU season can facilitates rapid coral adaptation to the dynamic upwelling environment (Zhu et al., 2023b). The transplanted coral has higher complexity and stability in addition to the AM network in July compared with the natural population, coral hosts with highly connected microbiomes are potentially more robust to environmental perturbations. Similarly, the coral microbiome from the inner reef zone exposed to stressful temperature fluctuations, potentially confers resilience to environmental disturbances, whereas host-associated microbial network in the outer reef with a more stable environment exhibits tightly connected interactions (Lima et al., 2020).
The network complexity and stability in source colonies of A. microphthalma were higher than those of A. hyacinthus throughout the two sampling periods. Another study has also observed significant variation in microbial networks across coral species, showing compartmentalization based on coral host identity (Dunphy et al., 2019). These patterns may be attributed to the successive vertical transmission of specific bacterial populations, leading to correlations (Leite et al., 2018b). Interestingly, we found that differences in the bacterial networks of transplanted corals between species were less pronounced in July, aligning with similar bacterial diversity and community between coral species. In the current study, bacterial communities of two coral species in a more homogeneous nursery environment tended to occupy a similar biological niche and interaction (Freilich et al., 2011; Lu et al., 2022). Additionally, it is believed that higher stability of the coral microbiome is associated with a greater complexity of interactions, making them less susceptible to microbial invasion or switching (Leite et al., 2018a; Mallon et al., 2015).
Understanding the assembly mechanisms that shape microbial community is a key topic in ecology (Nemergut et al., 2013; Stegen et al., 2012), but little is known about the assembly processes of coral microbial communities (Price et al., 2021). In this study, the ecological assembly processes of bacterial communities showed significant differences between May and July, while there was a consistent assembly mechanism for different coral species and transplanted corals. Specifically, stochastic process shaped the bacterial community of all corals in May, while deterministic process dominated the assembly process in July. A previous study also suggested that niche-based deterministic selection dominated the bacterial community assembly in corals during the upwelling season, where upwelling interference, sensitive network structures and closer bacterial associations might partly explain this observation (Zhu et al., 2023b). Stochastic processes are hypothesized to play a more critical role in microbial communities associated with corals under environmental stress, such as elevated temperatures and nutrient pollution (Zaneveld et al., 2016, 2017). However, it is worth noting that stochastic processes can also been observed in presumably healthy corals (Price et al., 2021; Zhu et al., 2022b). Therefore, both the bacterial communities of source colonies and transplanted Acropora corals were responsive to seasonal environmental fluctuations, contributing to the different community assembly processes.
Understanding the link between coral microbiome and environmental changes is essential for underpinning effective management and restoration strategies (Glasl et al., 2017). More importantly, we correlated the bacterial dynamic response of transplanted fragments to the marine environmental parameters in the area where the nursery was located. Among the detected environmental factors, our study revealed that physical variable (e.g., temperature) and nutrient condition (e.g., ammonium) played key roles in the shifts of coral associated bacterial communities. Water quality has been proved to be the important environmental drivers in controlling the coral microbiome (McDevitt-Irwin et al., 2017). For example, seawater temperature and nutrient enrichment can significantly affect the coral-associated bacterial communities from the Hainan Island (Zhu et al., 2023a, 2023b). Corals with environmental flexibility that adjust microbial composition to offset possible negative dysbiosis will be 'winners' from climate change (Röthig et al., 2020; Ziegler et al., 2019). In fact, we found all coral samples from transplanted fragments and source colonies were macroscopically healthy, indicating that microbiome flexibility is a key mechanism for coral to tolerate the environmental pressure (Deignan et al., 2023; Voolstra and Ziegler, 2020). Knowledge about the dynamic response of coral microbiome to specific environmental variables is essential for selecting the most appropriate areas and conservation methods.
5. Conclusions
In summary, our study characterized bacterial temporal dynamics of two Acropora coral hosts between transplanted fragments and source colonies including the community composition, co-occurrence pattern and assembly process. We found that seasonal environmental fluctuations significantly influenced the bacterial composition, assembly, and network, indicating that the microbiome of nursery-planting coral fragments showed similar flexibility to that of corals on source reefs under regional environmental scale. The critical bacterial assemblages and assembly mechanisms between the transplants and source colonies became similar over time for corresponding coral species, with transient changes in bacterial diversity and interaction network attributed to the effects of nursery frames. Furthermore, microbial communities associated with coral species of source colonies persisted high host specificity, while transplanted fragments in nursery had similar bacterial diversity, community structure and core microbiome across coral species, indicating that in situ coral nursery caused microbial community homogenization in transplants. Seawater temperature and ammonium concentration played key roles in the compositional shifts of bacterial communities associated with the transplanted fragments and source colonies. Finally, our findings suggested that shifts and homogeneity in bacterial communities of commonly transplanted Acropora corals might be adaptations to environmental perturbations and the nursery-planting process during short-term transplantation. Nevertheless, long-term microbial monitoring of transplanted fragments is needed to understand how they help corals adapt to the nursery environment.
Author Contributions
Wentao Zhu: Conceptualization, Investigation, Metodology, Writing – original draft. Zhuoran Li, Junling Zhang, Xiangbo Liu, Hao Wang, Rouwen Chen: Investigation. Xiubao Li: conceptualization, Writing – review & editing, Supervision.
-
Figure 1. Experimental design and sampling timeline during the nursery phase. In-situ coral nursery frame that transplanted coral fragments of Acropora hyacinthus and Acropora microphthalma were built in the degraded area adjacent to the reef in Fenjiezhou Island. Acropora fragments were originally collected from source colonies on the reef, and then each fragment was based to the nursery frame using a cable tie. For a sampling event (in May and July) during of the experiment, six small partial fragments of each species were obtained from the coral nursery and the six corresponding source colonies of each species were sampled.
Figure 3. CPCoA (Bray–Curtis) plot of bacterial associations within coral host habitats of two Acropora corals (a). The profiles of bacterial community associated with two Acropora corals in May (b) and July (c) between nursery fragments and source colonies. Bacterial community composition (relative abundances) at the family level of nursery fragments and source colonies in May and July (d).
Figure 5. Co-occurrence networks (a) and topological properties (b) of coral microbiomes for two Acropora corals collected from nursery fragments and source colonies in May and July. Node color represents different abundant bacterial OTUs within each network. The size of the nodes indicates the number of connections (degree) for each node. Connection between two nodes represents correlations, positive and negative correlations are shown in blue or red lines, respectively.
Figure 6. Fit of the neutral community model (a) and comparison of modified stochasticity ratio (b) showing ecological processes that shape the bacterial community assembly for two Acropora corals collected from nursery fragments and source colonies in May and July. The solid orange line is the best fit to the neutral community model, and the dashed orange line indicates 95% confidence intervals around the model prediction. OTUs that occur more or less frequently than predicted by the model are shown in grey and brown, respectively. R2 represents the goodness of fit to the model. Redundancy analysis (RDA) of coral-associated bacterial community dynamics with environmental variables (c).
-
Abrina T A S, Bennett J. 2021. A benefit-cost comparison of varying scales and methods of coral reef restoration in the Philippines. Science of the Total Environment, 799: 149325, doi: 10.1016/j.scitotenv.2021.149325 Aguirre E G, Million W C, Bartels E, et al. 2022. Host-specific epibiomes of distinct Acropora cervicornis genotypes persist after field transplantation. Coral Reefs, 41(2): 265–276, doi: 10.1007/s00338-022-02218-x Ainsworth T D, Krause L, Bridge T, et al. 2015. The coral core microbiome identifies rare bacterial taxa as ubiquitous endosymbionts. The ISME Journal, 9(10): 2261–2274, doi: 10.1038/ismej.2015.39 Beatty D S, Clements C S, Valayil J M, et al. 2022. Variance of coral anti-pathogen defense in response to transplantation between coral- and macroalgal-dominated reefs. Coral Reefs, 41(5): 1417–1431, doi: 10.1007/s00338-022-02291-2 Bourne D G, Morrow K M, Webster N S. 2016. Insights into the coral microbiome: underpinning the health and resilience of reef ecosystems. Annual Review of Microbiology, 70(1): 317–340, doi: 10.1146/annurev-micro-102215-095440 Brener-Raffalli K, Clerissi C, Vidal-Dupiol J, et al. 2018. Thermal regime and host clade, rather than geography, drive Symbiodinium and bacterial assemblages in the scleractinian coral Pocillopora damicornis sensu lato. Microbiome, 6(1): 39, doi: 10.1186/s40168-018-0423-6 Cárdenas A, Rodriguez-R L M, Pizarro V, et al. 2012. Shifts in bacterial communities of two caribbean reef-building coral species affected by white plague disease. The ISME Journal, 6(3): 502–512, doi: 10.1038/ismej.2011.123 Ceh J, van Keulen M, Bourne D G. 2013. Intergenerational transfer of specific bacteria in corals and possible implications for offspring fitness. Microbial Ecology, 65(1): 227–231, doi: 10.1007/s00248-012-0105-z Damjanovic K, Blackall L L, Peplow L M, et al. 2020. Assessment of bacterial community composition within and among Acropora loripes colonies in the wild and in captivity. Coral Reefs, 39(5): 1245–1255, doi: 10.1007/s00338-020-01958-y Deignan L K, McDougald D. 2022. Differential response of the microbiome of Pocillopora acuta to reciprocal transplantation within Singapore. Microbial Ecology, 83(3): 608–618, doi: 10.1007/s00248-021-01793-w Deignan L K, Pwa K H, Loh A A R, et al. 2023. The microbiomes of two Singaporean corals show site-specific differentiation and variability that correlates with the seasonal monsoons. Coral Reefs, 42(3): 677–691, doi: 10.1007/s00338-023-02376-6 Dunphy C M, Gouhier T C, Chu N D, et al. 2019. Structure and stability of the coral microbiome in space and time. Scientific Reports, 9(1): 6785, doi: 10.1038/s41598-019-43268-6 Engelen A H, Aires T, Vermeij M J A, et al. 2018. Host differentiation and compartmentalization of microbial communities in the azooxanthellate cupcorals Tubastrea coccinea and Rhizopsammia goesi in the Caribbean. Frontiers in Marine Science, 5: 391, doi: 10.3389/fmars.2018.00391 Freilich S, Zarecki R, Eilam O, et al. 2011. Competitive and cooperative metabolic interactions in bacterial communities. Nature Communications, 2(1): 589, doi: 10.1038/ncomms1597 Glasl B, Webster N S, Bourne D G. 2017. Microbial indicators as a diagnostic tool for assessing water quality and climate stress in coral reef ecosystems. Marine Biology, 164(4): 91, doi: 10.1007/s00227-017-3097-x Hernández-Zulueta J, Díaz-Pérez L, García-Maldonado J Q, et al. 2022. Bacterial assemblages associated with Acropora palmata affected by white band disease in the Mexican region of the Caribbean and Gulf of Mexico. Journal of Sea Research, 185: 102230, doi: 10.1016/j.seares.2022.102230 Houk P, Castro F, McInnis A, et al. 2022. Nutrient thresholds to protect water quality, coral reefs, and nearshore fisheries. Marine Pollution Bulletin, 184: 114144, doi: 10.1016/j.marpolbul.2022.114144 Hughes T P, Barnes M L, Bellwood D R, et al. 2017. Coral reefs in the Anthropocene. Nature, 546(7656): 82–90, doi: 10.1038/nature22901 Kanisan D P, Quek Z B R, Oh R M, et al. 2023. Diversity and distribution of microbial communities associated with reef corals of the Malay Peninsula. Microbial Ecology, 85(1): 37–48, doi: 10.1007/s00248-022-01958-1 Kellogg C A. 2019. Microbiomes of stony and soft deep-sea corals share rare core bacteria. Microbiome, 7(1): 90, doi: 10.1186/s40168-019-0697-3 Lee O O, Yang Jiangke, Bougouffa S, et al. 2012. Spatial and species variations in bacterial communities associated with corals from the red sea as revealed by pyrosequencing. Applied and Environmental Microbiology, 78(20): 7173–7184, doi: 10.1128/AEM.01111-12 Leite D C A, Salles J F, Calderon E N, et al. 2018a. Coral bacterial-core abundance and network complexity as proxies for anthropogenic pollution. Frontiers in Microbiology, 9: 833, doi: 10.3389/fmicb.2018.00833 Leite D C A, Salles J F, Calderon E N, et al. 2018b. Specific plasmid patterns and high rates of bacterial co-occurrence within the coral Holobiont. Ecology and Evolution, 8(3): 1818–1832, doi: 10.1002/ece3.3717 Lema K A, Bourne D G, Willis B L. 2014. Onset and establishment of diazotrophs and other bacterial associates in the early life history stages of the coral Acropora millepora. Molecular Ecology, 23(19): 4682–4695, doi: 10.1111/mec.12899 Lima L F O, Weissman M, Reed M, et al. 2020. Modeling of the coral microbiome: the influence of temperature and microbial network. mBio, 11(2): e02619–e02691, doi: 10.1128/mBio.02691-19 Liu Xiangbo, Zhu Wentao, Chen Ruimei, et al. 2024. Framed reef modules: a new and cost-effective tool for coral restoration. Restoration Ecology, 32(1): e13997, doi: 10.1111/rec.13997 Lu Meiqing, Wang Xuejing, Li Hailong, et al. 2022. Microbial community assembly and co-occurrence relationship in sediments of the river-dominated estuary and the adjacent shelf in the wet season. Environmental Pollution, 308: 119572, doi: 10.1016/j.envpol.2022.119572 Mallon C A, van Elsas J D, Salles J F. 2015. Microbial invasions: the process, patterns, and mechanisms. Trends in Microbiology, 23(11): 719–729, doi: 10.1016/j.tim.2015.07.013 McDevitt-Irwin J M, Baum J K, Garren M, et al. 2017. Responses of coral-associated bacterial communities to local and global stressors. Frontiers in Marine Science, 4: 262, doi: 10.3389/fmars.2017.00262 Miller N, Maneval P, Manfrino C, et al. 2020. Spatial distribution of microbial communities among colonies and genotypes in nursery-reared Acropora cervicornis. PeerJ, 8: e9635, doi: 10.7717/peerj.9635 Moberg F, Folke C. 1999. Ecological goods and services of coral reef ecosystems. Ecological Economics, 29(2): 215–233, doi: 10.1016/S0921-8009(99)00009-9 Morrow K M, Pankey M S, Lesser M P. 2022. Community structure of coral microbiomes is dependent on host morphology. Microbiome, 10(1): 113, doi: 10.1186/s40168-022-01308-w Nemergut D R, Schmidt S K, Fukami T, et al. 2013. Patterns and processes of microbial community assembly. Microbiology and Molecular Biology Reviews, 77(3): 342–356, doi: 10.1128/MMBR.00051-12 Ning Daliang, Deng Ye, Tiedje J M, et al. 2019. A general framework for quantitatively assessing ecological stochasticity. Proceedings of the National Academy of Sciences of the United States of America, 116(34): 16892–16898, doi: 10.1073/pnas.1904623116 Osman E O, Suggett D J, Voolstra C R, et al. 2020. Coral microbiome composition along the northern red sea suggests high plasticity of bacterial and specificity of endosymbiotic dinoflagellate communities. Microbiome, 8(1): 8, doi: 10.1186/s40168-019-0776-5 Peixoto R S, Rosado P M, de Assis Leite D C, et al. 2017. Beneficial microorganisms for corals (BMC): proposed mechanisms for coral health and resilience. Frontiers in Microbiology, 8: 341 Price J T, McLachlan R H, Jury C P, et al. 2021. Effect of species, provenance, and coral physiology on the composition of Hawaiian coral-associated microbial communities. Coral Reefs, 40(5): 1537–1548, doi: 10.1007/s00338-021-02164-0 Ramesh C H, Koushik S, Shunmugaraj T, et al. 2020. Seasonal studies on in situ coral transplantation in the Gulf of Mannar Marine Biosphere Reserve, Southeast coast of Tamil Nadu, India. Ecological Engineering, 152: 105884, doi: 10.1016/j.ecoleng.2020.105884 Randall C J, Toth L T, Leichter J J, et al. 2020. Upwelling buffers climate change impacts on coral reefs of the eastern tropical pacific. Ecology, 101(2): e02918, doi: 10.1002/ecy.2918 Reshef L, Koren O, Loya Y, et al. 2006. The coral probiotic hypothesis. Environmental Microbiology, 8(12): 2068–2073, doi: 10.1111/j.1462-2920.2006.01148.x Ricci F, Tandon K, Black J R, et al. 2022. Host traits and phylogeny contribute to shaping coral-bacterial symbioses. mSystems, 7(2): e00044–22, doi: 10.1128/msystems.00044-22 Rinkevich B. 2005. Conservation of coral reefs through active restoration measures: recent approaches and last decade progress. Environmental Science & Technology, 39(12): 4333–4342, doi: 10.1021/es0482583 Rinkevich B. 2021. Augmenting coral adaptation to climate change via coral gardening (the nursery phase). Journal of Environmental Management, 291: 112727, doi: 10.1016/j.jenvman.2021.112727 Röthig T, Bravo H, Corley A, et al. 2020. Environmental flexibility in Oulastrea crispata in a highly urbanised environment: a microbial perspective. Coral Reefs, 39(3): 649–662, doi: 10.1007/s00338-020-01938-2 Sloan W T, Lunn M, Woodcock S, et al. 2006. Quantifying the roles of immigration and chance in shaping prokaryote community structure. Environmental Microbiology, 8(4): 732–740, doi: 10.1111/j.1462-2920.2005.00956.x Smith K M, Payton T G, Sims R J, et al. 2019. Impacts of consecutive bleaching events and local algal abundance on transplanted coral colonies in the Florida keys. Coral Reefs, 38(4): 851–861, doi: 10.1007/s00338-019-01823-7 Stegen J C, Lin Xueju, Konopka A E, et al. 2012. Stochastic and deterministic assembly processes in subsurface microbial communities. The ISME Journal, 6(9): 1653–1664, doi: 10.1038/ismej.2012.22 Strudwick P, Seymour J, Camp E F, et al. 2022. Impacts of nursery-based propagation and out-planting on coral-associated bacterial communities. Coral Reefs, 41(1): 95–112, doi: 10.1007/s00338-021-02207-6 van Bleijswijk J D L, Whalen C, Duineveld G C A, et al. 2015. Microbial assemblages on a cold-water coral mound at the SE Rockall Bank (NE Atlantic): interactions with hydrography and topography. Biogeosciences, 12(14): 4483–4496, doi: 10.5194/bg-12-4483-2015 Voolstra C R, Ziegler M. 2020. Adapting with microbial help: microbiome flexibility facilitates rapid responses to environmental change. BioEssays, 42(7): 2000004, doi: 10.1002/bies.202000004 Webster N S, Reusch T B H, 2017. Microbial contributions to the persistence of coral reefs. The ISME Journal, 11(10): 2167-2174, doi: 10.1038/ismej.2017.66 Williams S L, Sur C, Janetski N, et al. 2019. Large-scale coral reef rehabilitation after blast fishing in Indonesia. Restoration Ecology, 27(2): 447–456, doi: 10.1111/rec.12866 Xia Jingquan, Zhu Wentao, Liu Xiangbo, et al. 2022. The effect of two types of grid transplantation on coral growth and the in-situ ecological restoration in a fragmented reef of the South China sea. Ecological Engineering, 177: 106558, doi: 10.1016/j.ecoleng.2022.106558 Zaneveld J R, Burkepile D E, Shantz A A, et al. 2016. Overfishing and nutrient pollution interact with temperature to disrupt coral reefs down to microbial scales. Nature Communications, 7(1): 11833, doi: 10.1038/ncomms11833 Zaneveld J R, McMinds R, Vega Thurber R, 2017. Stress and stability: applying the Anna Karenina principle to animal microbiomes. Nature Microbiology, 2(9): 17121, doi: 10.1038/nmicrobiol.2017.121 Zanotti A A, Gregoracci G B, Capel K C C, et al. 2020. Microbiome of the Southwestern Atlantic invasive scleractinian coral, Tubastraea tagusensis. Animal Microbiome, 2(1): 29, doi: 10.1186/s42523-020-00047-3 Zhu Wentao, Liu Xiangbo, Zhu Ming, et al. 2022b. Responses of symbiodiniaceae shuffling and microbial community assembly in thermally stressed Acropora hyacinthus. Frontiers in Microbiology, 13: 832081, doi: 10.3389/fmicb.2022.832081 Zhu Wentao, Liu Xiangbo, Zhang Junling, et al. 2023a. Response of coral bacterial composition and function to water quality variations under anthropogenic influence. Science of the Total Environment, 884: 163837, doi: 10.1016/j.scitotenv.2023.163837 Zhu Wentao, Liu Xiangbo, Zhang Junling, et al. 2023a. Response of coral bacterial composition and function to water quality variations under anthropogenic influence. Science of the Total Environment, 884: 163837, doi: 10.1016/j.scitotenv.2023.163837 (查阅网上资料,本条文献与上条文献重复,请确认) Zhu Wentao, Liu Xiangbo, Zhu Ming, et al. 2023b. Coastal upwelling under anthropogenic influence drives the community change, assembly process, and co-occurrence pattern of coral associated microorganisms. Journal of Geophysical Research: Oceans, 128(2): e2022JC019307, doi: 10.1029/2022JC019307 Zhu Wentao, Ren Yuxiao, Liu Xiangbo, et al. 2022a. The impact of coastal upwelling on coral reef ecosystem under anthropogenic influence: coral reef community and its response to environmental factors. Frontiers in Marine Science, 9: 888888, doi: 10.3389/fmars.2022.888888 Ziegler M, Grupstra C G B, Barreto M M, et al. 2019. Coral bacterial community structure responds to environmental change in a host-specific manner. Nature Communications, 10(1): 3092, doi: 10.1038/s41467-019-10969-5 Ziegler M, Seneca F O, Yum L K, et al. 2017. Bacterial community dynamics are linked to patterns of coral heat tolerance. Nature Communications, 8(1): 14213, doi: 10.1038/ncomms14213 -
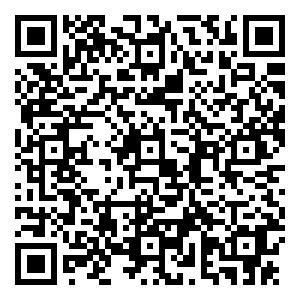
计量
- 文章访问数: 20
- HTML全文浏览量: 8
- 被引次数: 0